The Advance of Conventional Drilling
I've previously written about improvements in geothermal drilling technology and strategies to overcome its weakness in producing electricity. Geothermal is challenging because the wells must be extremely deep (and hot) to improve energy production and increase power plant efficiency. My main conclusions were that drillers could adapt conventional techniques to reach 60,000' depths and that selling process heat would be more profitable than producing electricity.
Drilling Progress Highlights
There have been many positive developments in the last 18 months:
-
The Utah FORGE project continues to improve its drilling speed and bit runs. They confirmed that plain water is a superior drilling fluid for granite. Penetration rate increased while bit life improved.
-
The startup Eavor drilled a test well called "Eavor Deep" in New Mexico that reached a bottom hole temperature of 250 C, earning $20 million in progress payments.
-
The Haynesville Shale has been active post-COVID and has a relatively high bottom-hole temperature. Drilling motor companies have put in the effort to increase the temperature tolerance of their motors, allowing operators to get longer runs and ditch rentals like mud coolers.
-
Higher temperature directional tools seemed far away, but now giants like Oxy and startups with blue chip talent like XGS think tools that can drill in rock up to 300 C are near commercialization.
Implications
These developments make my drilling proposal unnecessary. The purpose was to prove that conventional drilling could drill at acceptable penetration rates in the granite, that there were possible architectures that didn't need high-temperature directional tools, and that drilling costs might increase sublinearly in non-sedimentary rock. Those points are now proven or obsoleted. The focus shifts from demonstrating technology to creating a business.
Technology Demonstration vs. Making Money
Turning my proposal into a business is not impossible, but it has some challenges. The most significant is that existing drilling rigs can only reach ~10 km deep. A vertical, closed-loop well only 10 km deep would struggle to beat natural gas for process heat except in a few locations with steep temperature gradients. And even those areas may not work at sub $3/MCF gas. There isn't enough heat transfer area. A new drilling rig for deep drilling might cost $40 million.
Another issue I've discussed before is limited defensibility with conventional drilling technology. Vendors own all the technology, and the operator is a project developer. Acreage is worth very little since hot, dry rock is ubiquitous. Returns will be closer to a solar developer than an oil and gas company or a tech startup. Project development can still be a good business, but it requires financing to juice returns, and lenders want low-risk projects. There is a needle to thread where the first few projects will be tough to finance, and being the first won't lead to massive appreciation in acreage prices.
A final wrinkle is that process heat is not a fungible commodity. One cubic foot of natural gas is as good as another, and there is an existing transport network. An engineer at a factory will size a natural gas boiler for their needs and open the gas valve when it's time to run. Providing geothermal process heat is more like selling a boiler. It requires a much closer and more collaborative relationship with the customer.
Companies need a coherent strategy to deal with these issues.
Eavor's Solutions
Eavor uses closed-loop wellbores to heat fluid (see picture below). The technical and regulatory risk is minimal compared to enhanced geothermal (EGS) concepts, where fluid is pumped from an injection well through the reservoir to a producer well. Drilling is underway for the first commercial project in Germany. There are several takeaways important for earning money from geothermal projects:
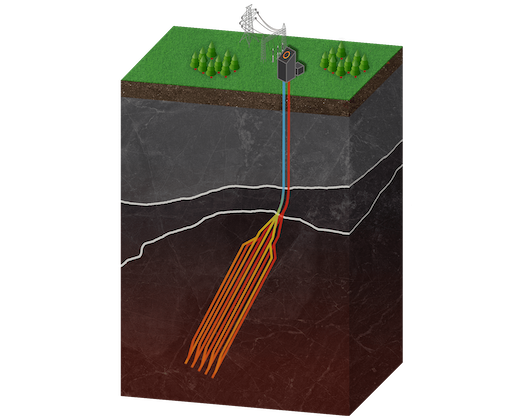
Source: eavor.com
-
Almost Geothermal Anywhere
The concept works everywhere except for deep sedimentary basins. Fracking bans, seismic activity, and poor fracture connectivity are not barriers. The probability of having acceptable geology below potential customers for process heat is high. Technologies with more limitations usually sell electricity due to limited matching with more profitable customers.
-
Better Bankability
The thermal output of an Eavor "loop" is very predictable, and technical complexity is low. Lenders will be more comfortable with these projects than enhanced geothermal ones. The success rate for EGS has been poor, and every project carries technical risk (short-circuiting between wells, lack of connectivity) and regulatory risk (induced seismicity). Startups pursuing EGS will struggle to retire this risk. Geology is fickle with fracturing and seismicity. The ability to borrow at good terms is critical for geothermal projects because of their high upfront costs and the need for debt to juice equity returns.
-
Good Enough Bottom Hole Temperatures
What is the minimum bottom hole temperature that allows a project to be successful? It depends! Increasing bottom hole temperature is critical for electricity generation because high fluid temperature increases power plant efficiency and decreases capital expenditure per watt. Process heat applications are less sensitive to bottom-hole temperature because they have better efficiency. The bottom hole temperature still must exceed the required process temperature by 25-50C.
The Eavor Deep well proved 250C is possible. That is adequate for steam users like paper mills, lumber mills, and food processors. And current drilling rigs can't drill deep enough to reach rock exceeding 250C in most of the US. Using multiple laterals to increase heat transfer area in shallower wells is more economical than drilling more vertical wells because the "fixed costs" of locations, surface casing, and drilling the sedimentary portion of the well make up a large share of shallow vertical well cost. A vertical well needs to be much deeper to gain a cost advantage.
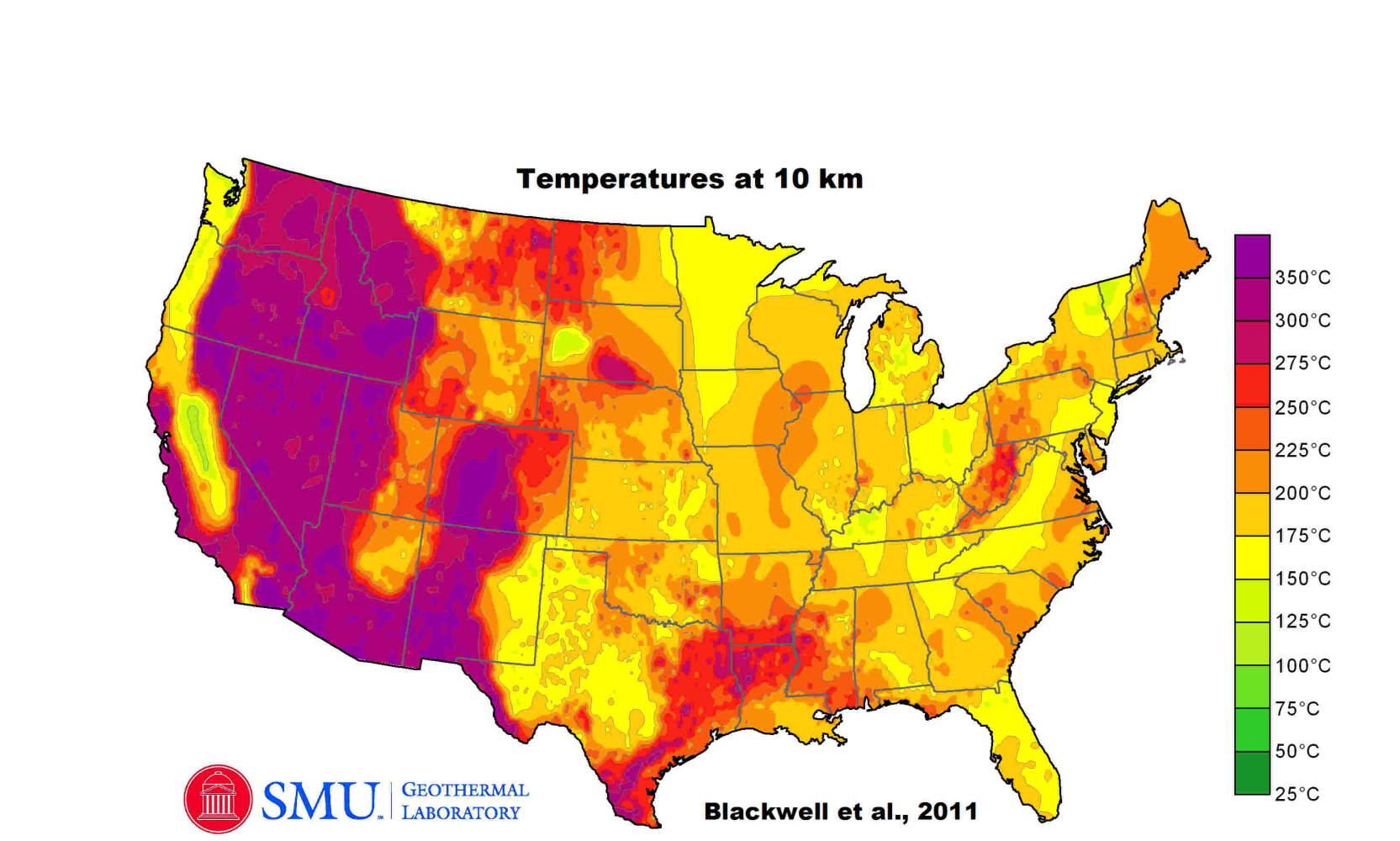
Source: SMU
The efficiency is low and cost high when Eavor does try to produce electricity. Their goal is only $60/MWh. That works fine in Germany because subsidies for geothermal exceed $200/MWh. But their wells can produce process heat 10x cheaper than electricity per unit of energy. Most of the company's European orders are district heating projects. Eavor can produce expensive electricity where there are subsidies or high prices and compete with natural gas on low-temperature process heat. Beating US shale gas someday is not out of the question because $10/MWh process heat equates to ~$3/MCF gas.
The upshot is that Eavor has a legitimate business that should enable new projects.
Starting the Flywheel
A small startup like Eavor can accomplish more than it seems because of how the oil and gas industry is structured. Two features trigger broad-based improvements:
-
Incremental Improvements Add Up
The oil and gas drilling industry is an excellent example of "learning by doing." Equipment like bits, motors, and directional tools are inspected after every run to determine the cause of failure. Service companies stop by the rig daily to record conditions and discuss the situation with the site supervisor. They transmit that feedback immediately to research and development. Bit companies optimize manufacturing for small batches. A bit design might have as few as fifty copies before the next one takes over. But the system only works if there is active drilling to drive iteration. Projects worth developing are a requirement to start.
Eavor tested several incremental improvements like insulated drill pipe paired and mud coolers. The drill string acts like a pipe-in-pipe heat exchanger as fluid circulates. The fluid has a relatively steady temperature between the bottom hole temperature and the surface temperature. Insulated drill pipe allows cool fluid to reach the directional tools and motor, preventing overheating. You can drill rock hotter than the equipment rating.
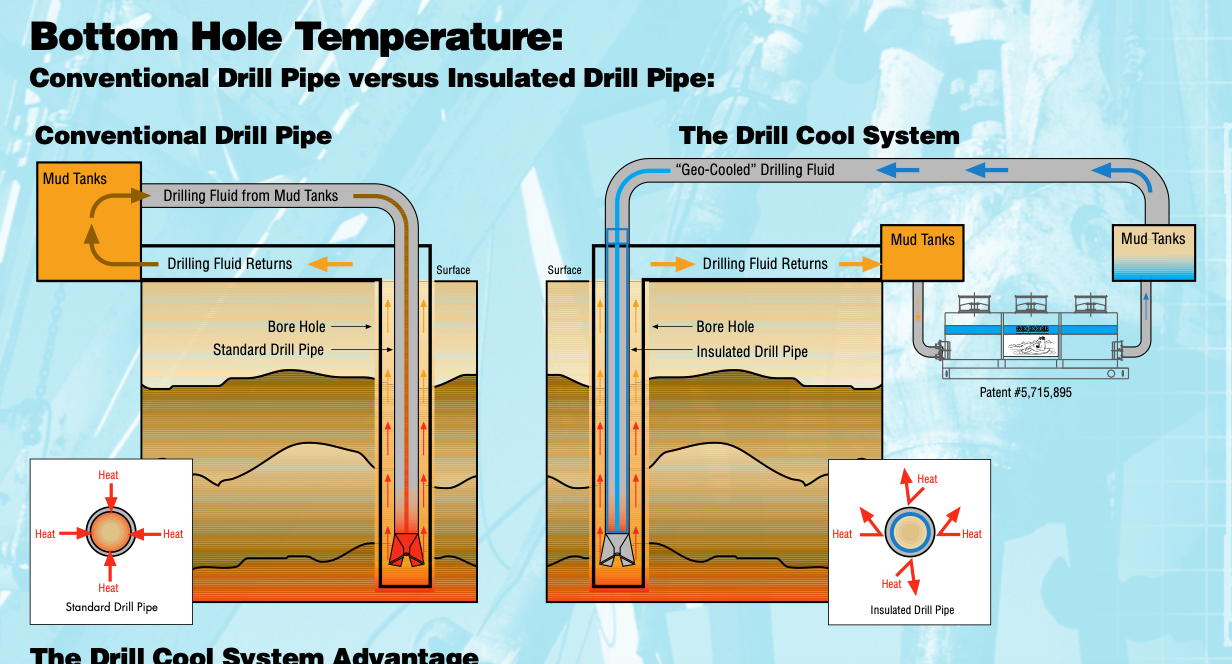
Source: Drill Cool Inc.
-
There is No Intellectual Property in Drilling
Oil companies focus on finding the best acreage and exploiting its value. Rarely do they own drilling rigs or drilling technology. Governments release well-level production data. Logs quickly enter the public domain. No one can distort their results for long. Service companies happily provide detailed information like bit records. A competent engineer could find all of Eavor's key vendors within a few hours. A company like Eavor might try to "tight hole" its vendors from sharing data, but it can't keep them from using their process knowledge to create detailed proposals and win more work.
Eavor claims it has proprietary technology in things like gunk to seal fractures (eliminating the need for casing) or using insulated drill pipe. But there is someone they buy that gunk from, and you can rent insulated drill pipe. The company's funding makes it look like a mix of a project developer and paid technology demonstrator. It collected $20 million from BP and H&P (drilling rigs) for meeting technical goals on its Eavor Deep well. These companies would benefit from new geothermal technology because it would mean more project development opportunities and increased demand for drilling rigs.
Eavor may or may not be a good investment. But they don't need to produce VC-like returns to help birth a new phase for the geothermal industry. Simply developing projects and starting the flywheel will suffice. Many in the industry will provide funding to give them that shot. Fast followers can emerge from the woodwork once the concept is proven.
Breaking the Obsession with Permeability
Eavor only uses conduction in the reservoir to move heat. Most startups use in-reservoir convection to increase heat flows and decrease drilling footage. Usually, that means enhanced geothermal, but there are also hybrid options where the aim is to induce convection in the reservoir without using open-loop circulation. Oil and gas wells must have permeability because they are trying to remove hydrocarbons. Geothermal wells don't. Moving fluids through reservoirs comes with complexity, unpredictability, and challenges that conduction does not. There is room for improvement with conduction because the thermal conductivity of granite is awful. Metals like copper or aluminum conduct heat 100x better.
I've thought about "fracking-for-conduction" as a way to improve the performance of vertical wells, but the improvement isn't earth-shattering. XGS Energy is pursuing enhanced conduction in a directional well to improve performance. Operators have limited control over how a fracture propagates during hydraulic fracturing. The iron law is that fluid travels the path of least resistance. Pumping rate and volume impact fracture height and length, but the stress regime in the rock and paths of existing fractures determine fracture orientation. Fractures in deeper wells are vertical and have a favored direction. Oil companies know the stress regime ahead of time and drill perpendicular to the direction fractures will propagate. Vertical wells have limited numbers of fracture stages because they run parallel to the wellbore. A horizontal well with the correct orientation can have dozens, vastly increasing the impacted rock volume.
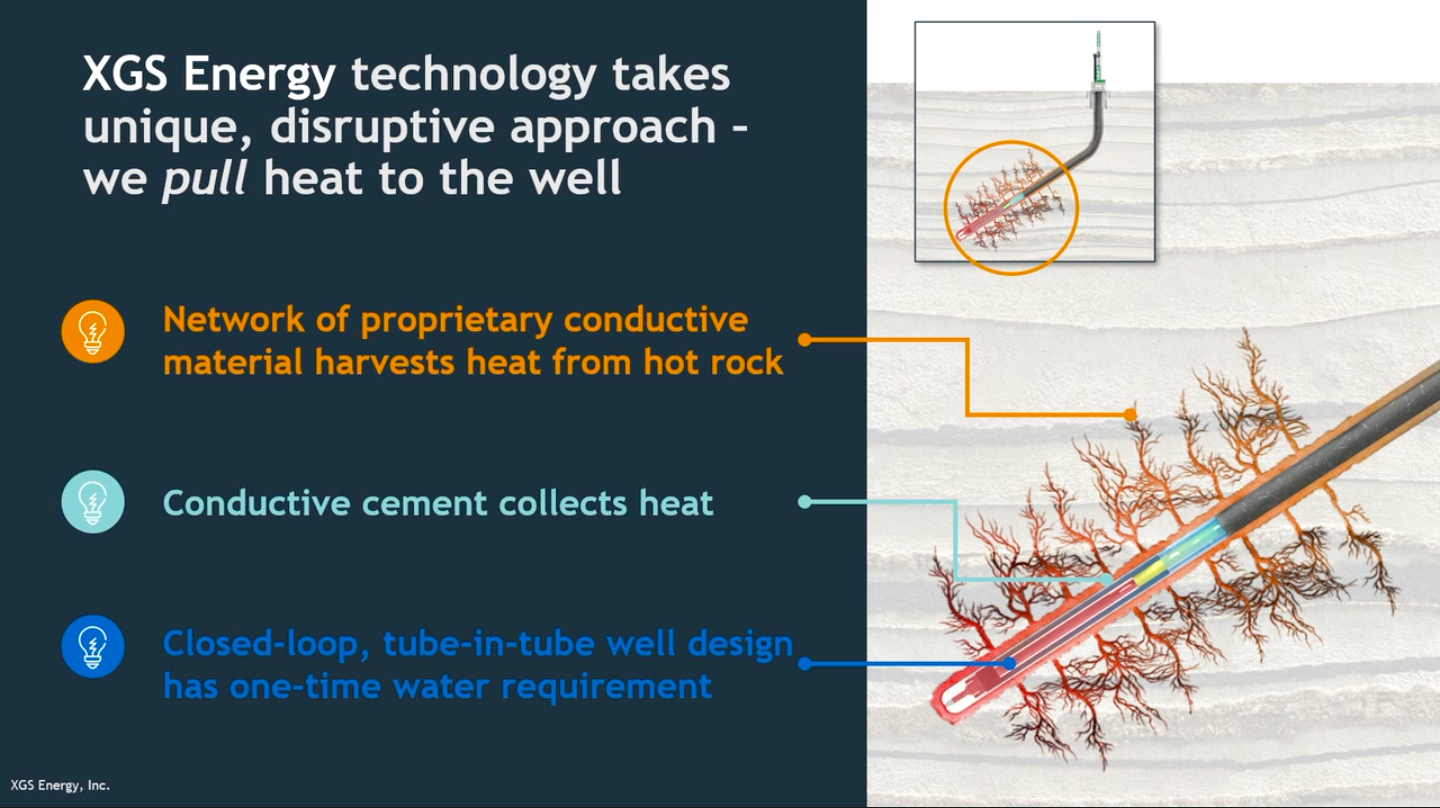
Source: XGS
XGS's concept is technologically conservative because it much more closely emulates multi-stage fractured horizontal oil and gas wells than enhanced geothermal projects in granite do (which are more like an attempt to water flood fractured reservoirs, yikes!). There are a few differences:
-
The Lateral is at a 45 Degree Inclination
The lateral for an oil and gas well must match the inclination of the geological formation to stay in the pay zone, but it is all pay in dry rock geothermal. An angled lateral can keep gaining depth (and temperature) while maintaining acceptable fracture geometry. It might even be possible to ditch directional tools after the curve if the bottom hole assembly can hold the inclination for an extended time. Bits have gotten much better at this over the years, and it might be feasible if paired with a stiff bottom-hole assembly.
-
Proppant is Replaced with Heat Conductive Slurry
Oil and gas wells must pack sand into fractures to prop them open and allow hydrocarbons to flow into the wellbore. Frac crews pump water to initiate fractures in the rock and carry the sand. XGS will replace sand with a material ~50x more conductive than granite (maybe aluminum pellets). Fractures will close and squash the material together to create a high-conductivity channel. The conductive slurry is the most significant technical risk, but the industry understands how particles like proppant disperse in fractures. And an important lesson from unconventional oil and gas wells is that distribution doesn't have to be perfect. "Good enough" paired with quantity works.
-
Cementing Happens After Stimulation
Normally cementing after fracking would destroy a well by blocking the fractures. In this case, cement helps to thermally connect the rock to the casing and displace free fluid with lower conductivity. Using casing is much more conservative than Eavor and reflects an ambition to use higher-pressure circulating fluid.
XGS and Eavor are both pursuing designs with low technical and regulatory risks that are highly repeatable and leverage oil and gas technology. XGS will have shorter, more expensive laterals that are more productive per foot than Eavor. The XGS architecture's goal is to reach 300C+ temperatures where conventional steam turbines become practical. The company has close ties to Baker Hughes, which built the one-off prototypes of directional tools and motors for drilling near volcanoes in Iceland. Shorter, hotter laterals would favor this more exotic technology where Eavor needs to use commodity equipment. XGS isn't listing cost targets, but $50/MWh for electricity might be a solid medium-term goal based on how I estimate their costs. Future cost reduction potential comes from faster drilling, longer and hotter laterals, more frac stages, better fracture length, and increased pipe diameter to accommodate a higher fluid flow.
These concepts compete but will also assist each other in developing the technology. Any improvement in drilling bits, motors, drilling rigs, or tools will help both companies. Any project that helps make lenders more comfortable with closed-loop geothermal systems will make the next one easier to finance. One technology may go extinct, or they might specialize in different applications.
Appraising the Competition
The rest of the world isn't standing still. Many companies are working towards cheaper ways to provide process heat and firm electricity.
My industrial process heat post covers how thermal storage in bricks is one of the more capable competitors. A leading company in the space recently announced a factory that will produce 90 GWh of storage per year. Current production is 2.4 GWh per year. Each Eavor and XGS project equals 1.3 GWh and 200 MWh on a comparable basis, respectively. Analysts estimate the solar PV supply chain will have hundreds of gigawatts of excess capacity this year. There is no shortage of energy to heat the bricks. Geothermal should have a lower potential cost but lacks the simplicity and flexibility of putting bricks in an insulated container. Most of the world has cheaper solar and more expensive drilling than the US, compounding these issues. The US has cheap natural gas because it has inexpensive drilling, making the US a tough home market.
Many models suggest grids will need more "clean, firm" power than they have now. Others prefer building more power lines to counteract variability in wind and solar ("the wind is always blowing somewhere"). And some companies just want to sell batteries. Transmission and batteries could substitute for "clean, firm" power plants like geothermal or nuclear if they are cheaper.
Most of the electricity transmission discourse focuses on long-distance, overhead power lines. These often face stiff opposition. But regional projects still add significant resiliency and face fewer challenges. Buried HVDC lines on existing right-of-way fly through approvals compared to overhead lines. These projects reduce the need for dispatchable power plants even if they don't replace them.
Batteries strengthen the grid by firming renewables and improving the operation of existing thermal power plants. Power (Watts) is often more important for grid stability than energy storage (watt-hours), especially if fossil fuels are legal. Batteries are already comparable to simple cycle natural gas power plants on a cost-per-watt basis. Increasing the use of alternative chemistries and co-locating batteries with solar farms promises to drop that cost further. Batteries can provide much of the power needed at critical moments and charge from solar, wind, nuclear, natural gas, coal, etc. Thermal power plants can run steadily to charge batteries when supply is tight instead of being forced to ramp up and down, reducing maintenance costs while increasing availability.
Eventually, falling solar prices and new battery chemistries like iron-air or sodium-ion could push fossil fuels further down the generation stack and reduce the demand for transmission. Solar farms might buy more panels than they need (increasing DC:AC ratio) to smooth output on cloudy days. Batteries could store more energy affordably when electricity prices are low. Any electricity market that can utilize "smart" charging for the growing fleet of electric vehicles will compound the battery advantage.
Rapidly scaling competitors are a threat if your solution relies on "learning by doing." Thermal storage, batteries, falling renewables prices, and legal fossil fuels reduce demand for geothermal process heat and electricity. Geothermal is in a race to break out from being a forever-niche energy source.
How Far Can It Go?
The geothermal technology flywheel is getting close to being self-sustaining. Commercial closed-loop projects should be operational within the next 2-3 years. They will spark copycats if they are successful. Companies pursuing other technologies can switch immediately by changing vendors and their well design, similar to how many solar thermal projects converted to photovoltaics.
Talent isn't an issue in North America or Europe because the oil industry constantly trains and sheds workers due to the market cycle. Geothermal companies can hoover up the laid-off and disillusioned. It could be an issue in the areas of the world with thin oilfield service markets.
Process heat should be more profitable than electricity, but companies love touting electricity because of the large addressable market. Electricity is trendy! There should be demand for repeatable, low-risk architectures in either application, but electricity will require subsidies or favorable market rules in most locations for now. I'd recommend caution to any lobbyists trying to alter electricity markets to favor zero carbon, dispatchable power plants with >90% percent capacity factors and onsite fuel. Other technologies might find geothermal power plants producing $50/MWh electricity with <2-year construction times hard to beat!
Having the chance to get in the game and hone your craft is all you can ask for. Geothermal looks like it will get that chance. That could drop the cost of delivering process heat precipitously while the power plant portion of capital expenditure would quickly dominate electricity production cost. Developers and service companies will incrementally improve depth and temperature to increase the addressable market. Competition looms from other carbon-free process heat technologies and renewables firming techniques. Only time will tell if geothermal can gain a foothold in providing heat or earn a double-digit share of the electricity market.
2023 Geothermal Update
2023 June 30 Twitter Substack See all postsDrilling technology progresses, but is it enough?
The Advance of Conventional Drilling
I've previously written about improvements in geothermal drilling technology and strategies to overcome its weakness in producing electricity. Geothermal is challenging because the wells must be extremely deep (and hot) to improve energy production and increase power plant efficiency. My main conclusions were that drillers could adapt conventional techniques to reach 60,000' depths and that selling process heat would be more profitable than producing electricity.
Drilling Progress Highlights
There have been many positive developments in the last 18 months:
The Utah FORGE project continues to improve its drilling speed and bit runs. They confirmed that plain water is a superior drilling fluid for granite. Penetration rate increased while bit life improved.
The startup Eavor drilled a test well called "Eavor Deep" in New Mexico that reached a bottom hole temperature of 250 C, earning $20 million in progress payments.
The Haynesville Shale has been active post-COVID and has a relatively high bottom-hole temperature. Drilling motor companies have put in the effort to increase the temperature tolerance of their motors, allowing operators to get longer runs and ditch rentals like mud coolers.
Higher temperature directional tools seemed far away, but now giants like Oxy and startups with blue chip talent like XGS think tools that can drill in rock up to 300 C are near commercialization.
Implications
These developments make my drilling proposal unnecessary. The purpose was to prove that conventional drilling could drill at acceptable penetration rates in the granite, that there were possible architectures that didn't need high-temperature directional tools, and that drilling costs might increase sublinearly in non-sedimentary rock. Those points are now proven or obsoleted. The focus shifts from demonstrating technology to creating a business.
Technology Demonstration vs. Making Money
Turning my proposal into a business is not impossible, but it has some challenges. The most significant is that existing drilling rigs can only reach ~10 km deep. A vertical, closed-loop well only 10 km deep would struggle to beat natural gas for process heat except in a few locations with steep temperature gradients. And even those areas may not work at sub $3/MCF gas. There isn't enough heat transfer area. A new drilling rig for deep drilling might cost $40 million.
Another issue I've discussed before is limited defensibility with conventional drilling technology. Vendors own all the technology, and the operator is a project developer. Acreage is worth very little since hot, dry rock is ubiquitous. Returns will be closer to a solar developer than an oil and gas company or a tech startup. Project development can still be a good business, but it requires financing to juice returns, and lenders want low-risk projects. There is a needle to thread where the first few projects will be tough to finance, and being the first won't lead to massive appreciation in acreage prices.
A final wrinkle is that process heat is not a fungible commodity. One cubic foot of natural gas is as good as another, and there is an existing transport network. An engineer at a factory will size a natural gas boiler for their needs and open the gas valve when it's time to run. Providing geothermal process heat is more like selling a boiler. It requires a much closer and more collaborative relationship with the customer.
Companies need a coherent strategy to deal with these issues.
Eavor's Solutions
Eavor uses closed-loop wellbores to heat fluid (see picture below). The technical and regulatory risk is minimal compared to enhanced geothermal (EGS) concepts, where fluid is pumped from an injection well through the reservoir to a producer well. Drilling is underway for the first commercial project in Germany. There are several takeaways important for earning money from geothermal projects:
Source: eavor.com
Almost Geothermal Anywhere
The concept works everywhere except for deep sedimentary basins. Fracking bans, seismic activity, and poor fracture connectivity are not barriers. The probability of having acceptable geology below potential customers for process heat is high. Technologies with more limitations usually sell electricity due to limited matching with more profitable customers.
Better Bankability
The thermal output of an Eavor "loop" is very predictable, and technical complexity is low. Lenders will be more comfortable with these projects than enhanced geothermal ones. The success rate for EGS has been poor, and every project carries technical risk (short-circuiting between wells, lack of connectivity) and regulatory risk (induced seismicity). Startups pursuing EGS will struggle to retire this risk. Geology is fickle with fracturing and seismicity. The ability to borrow at good terms is critical for geothermal projects because of their high upfront costs and the need for debt to juice equity returns.
Good Enough Bottom Hole Temperatures
What is the minimum bottom hole temperature that allows a project to be successful? It depends! Increasing bottom hole temperature is critical for electricity generation because high fluid temperature increases power plant efficiency and decreases capital expenditure per watt. Process heat applications are less sensitive to bottom-hole temperature because they have better efficiency. The bottom hole temperature still must exceed the required process temperature by 25-50C.
The Eavor Deep well proved 250C is possible. That is adequate for steam users like paper mills, lumber mills, and food processors. And current drilling rigs can't drill deep enough to reach rock exceeding 250C in most of the US. Using multiple laterals to increase heat transfer area in shallower wells is more economical than drilling more vertical wells because the "fixed costs" of locations, surface casing, and drilling the sedimentary portion of the well make up a large share of shallow vertical well cost. A vertical well needs to be much deeper to gain a cost advantage.
Source: SMU
The efficiency is low and cost high when Eavor does try to produce electricity. Their goal is only $60/MWh. That works fine in Germany because subsidies for geothermal exceed $200/MWh. But their wells can produce process heat 10x cheaper than electricity per unit of energy. Most of the company's European orders are district heating projects. Eavor can produce expensive electricity where there are subsidies or high prices and compete with natural gas on low-temperature process heat. Beating US shale gas someday is not out of the question because $10/MWh process heat equates to ~$3/MCF gas.
The upshot is that Eavor has a legitimate business that should enable new projects.
Starting the Flywheel
A small startup like Eavor can accomplish more than it seems because of how the oil and gas industry is structured. Two features trigger broad-based improvements:
Incremental Improvements Add Up
The oil and gas drilling industry is an excellent example of "learning by doing." Equipment like bits, motors, and directional tools are inspected after every run to determine the cause of failure. Service companies stop by the rig daily to record conditions and discuss the situation with the site supervisor. They transmit that feedback immediately to research and development. Bit companies optimize manufacturing for small batches. A bit design might have as few as fifty copies before the next one takes over. But the system only works if there is active drilling to drive iteration. Projects worth developing are a requirement to start.
Eavor tested several incremental improvements like insulated drill pipe paired and mud coolers. The drill string acts like a pipe-in-pipe heat exchanger as fluid circulates. The fluid has a relatively steady temperature between the bottom hole temperature and the surface temperature. Insulated drill pipe allows cool fluid to reach the directional tools and motor, preventing overheating. You can drill rock hotter than the equipment rating.
Source: Drill Cool Inc.
There is No Intellectual Property in Drilling
Oil companies focus on finding the best acreage and exploiting its value. Rarely do they own drilling rigs or drilling technology. Governments release well-level production data. Logs quickly enter the public domain. No one can distort their results for long. Service companies happily provide detailed information like bit records. A competent engineer could find all of Eavor's key vendors within a few hours. A company like Eavor might try to "tight hole" its vendors from sharing data, but it can't keep them from using their process knowledge to create detailed proposals and win more work.
Eavor claims it has proprietary technology in things like gunk to seal fractures (eliminating the need for casing) or using insulated drill pipe. But there is someone they buy that gunk from, and you can rent insulated drill pipe. The company's funding makes it look like a mix of a project developer and paid technology demonstrator. It collected $20 million from BP and H&P (drilling rigs) for meeting technical goals on its Eavor Deep well. These companies would benefit from new geothermal technology because it would mean more project development opportunities and increased demand for drilling rigs.
Eavor may or may not be a good investment. But they don't need to produce VC-like returns to help birth a new phase for the geothermal industry. Simply developing projects and starting the flywheel will suffice. Many in the industry will provide funding to give them that shot. Fast followers can emerge from the woodwork once the concept is proven.
Breaking the Obsession with Permeability
Eavor only uses conduction in the reservoir to move heat. Most startups use in-reservoir convection to increase heat flows and decrease drilling footage. Usually, that means enhanced geothermal, but there are also hybrid options where the aim is to induce convection in the reservoir without using open-loop circulation. Oil and gas wells must have permeability because they are trying to remove hydrocarbons. Geothermal wells don't. Moving fluids through reservoirs comes with complexity, unpredictability, and challenges that conduction does not. There is room for improvement with conduction because the thermal conductivity of granite is awful. Metals like copper or aluminum conduct heat 100x better.
I've thought about "fracking-for-conduction" as a way to improve the performance of vertical wells, but the improvement isn't earth-shattering. XGS Energy is pursuing enhanced conduction in a directional well to improve performance. Operators have limited control over how a fracture propagates during hydraulic fracturing. The iron law is that fluid travels the path of least resistance. Pumping rate and volume impact fracture height and length, but the stress regime in the rock and paths of existing fractures determine fracture orientation. Fractures in deeper wells are vertical and have a favored direction. Oil companies know the stress regime ahead of time and drill perpendicular to the direction fractures will propagate. Vertical wells have limited numbers of fracture stages because they run parallel to the wellbore. A horizontal well with the correct orientation can have dozens, vastly increasing the impacted rock volume.
Source: XGS
XGS's concept is technologically conservative because it much more closely emulates multi-stage fractured horizontal oil and gas wells than enhanced geothermal projects in granite do (which are more like an attempt to water flood fractured reservoirs, yikes!). There are a few differences:
The Lateral is at a 45 Degree Inclination
The lateral for an oil and gas well must match the inclination of the geological formation to stay in the pay zone, but it is all pay in dry rock geothermal. An angled lateral can keep gaining depth (and temperature) while maintaining acceptable fracture geometry. It might even be possible to ditch directional tools after the curve if the bottom hole assembly can hold the inclination for an extended time. Bits have gotten much better at this over the years, and it might be feasible if paired with a stiff bottom-hole assembly.
Proppant is Replaced with Heat Conductive Slurry
Oil and gas wells must pack sand into fractures to prop them open and allow hydrocarbons to flow into the wellbore. Frac crews pump water to initiate fractures in the rock and carry the sand. XGS will replace sand with a material ~50x more conductive than granite (maybe aluminum pellets). Fractures will close and squash the material together to create a high-conductivity channel. The conductive slurry is the most significant technical risk, but the industry understands how particles like proppant disperse in fractures. And an important lesson from unconventional oil and gas wells is that distribution doesn't have to be perfect. "Good enough" paired with quantity works.
Cementing Happens After Stimulation
Normally cementing after fracking would destroy a well by blocking the fractures. In this case, cement helps to thermally connect the rock to the casing and displace free fluid with lower conductivity. Using casing is much more conservative than Eavor and reflects an ambition to use higher-pressure circulating fluid.
XGS and Eavor are both pursuing designs with low technical and regulatory risks that are highly repeatable and leverage oil and gas technology. XGS will have shorter, more expensive laterals that are more productive per foot than Eavor. The XGS architecture's goal is to reach 300C+ temperatures where conventional steam turbines become practical. The company has close ties to Baker Hughes, which built the one-off prototypes of directional tools and motors for drilling near volcanoes in Iceland. Shorter, hotter laterals would favor this more exotic technology where Eavor needs to use commodity equipment. XGS isn't listing cost targets, but $50/MWh for electricity might be a solid medium-term goal based on how I estimate their costs. Future cost reduction potential comes from faster drilling, longer and hotter laterals, more frac stages, better fracture length, and increased pipe diameter to accommodate a higher fluid flow.
These concepts compete but will also assist each other in developing the technology. Any improvement in drilling bits, motors, drilling rigs, or tools will help both companies. Any project that helps make lenders more comfortable with closed-loop geothermal systems will make the next one easier to finance. One technology may go extinct, or they might specialize in different applications.
Appraising the Competition
The rest of the world isn't standing still. Many companies are working towards cheaper ways to provide process heat and firm electricity.
My industrial process heat post covers how thermal storage in bricks is one of the more capable competitors. A leading company in the space recently announced a factory that will produce 90 GWh of storage per year. Current production is 2.4 GWh per year. Each Eavor and XGS project equals 1.3 GWh and 200 MWh on a comparable basis, respectively. Analysts estimate the solar PV supply chain will have hundreds of gigawatts of excess capacity this year. There is no shortage of energy to heat the bricks. Geothermal should have a lower potential cost but lacks the simplicity and flexibility of putting bricks in an insulated container. Most of the world has cheaper solar and more expensive drilling than the US, compounding these issues. The US has cheap natural gas because it has inexpensive drilling, making the US a tough home market.
Many models suggest grids will need more "clean, firm" power than they have now. Others prefer building more power lines to counteract variability in wind and solar ("the wind is always blowing somewhere"). And some companies just want to sell batteries. Transmission and batteries could substitute for "clean, firm" power plants like geothermal or nuclear if they are cheaper.
Most of the electricity transmission discourse focuses on long-distance, overhead power lines. These often face stiff opposition. But regional projects still add significant resiliency and face fewer challenges. Buried HVDC lines on existing right-of-way fly through approvals compared to overhead lines. These projects reduce the need for dispatchable power plants even if they don't replace them.
Batteries strengthen the grid by firming renewables and improving the operation of existing thermal power plants. Power (Watts) is often more important for grid stability than energy storage (watt-hours), especially if fossil fuels are legal. Batteries are already comparable to simple cycle natural gas power plants on a cost-per-watt basis. Increasing the use of alternative chemistries and co-locating batteries with solar farms promises to drop that cost further. Batteries can provide much of the power needed at critical moments and charge from solar, wind, nuclear, natural gas, coal, etc. Thermal power plants can run steadily to charge batteries when supply is tight instead of being forced to ramp up and down, reducing maintenance costs while increasing availability.
Eventually, falling solar prices and new battery chemistries like iron-air or sodium-ion could push fossil fuels further down the generation stack and reduce the demand for transmission. Solar farms might buy more panels than they need (increasing DC:AC ratio) to smooth output on cloudy days. Batteries could store more energy affordably when electricity prices are low. Any electricity market that can utilize "smart" charging for the growing fleet of electric vehicles will compound the battery advantage.
Rapidly scaling competitors are a threat if your solution relies on "learning by doing." Thermal storage, batteries, falling renewables prices, and legal fossil fuels reduce demand for geothermal process heat and electricity. Geothermal is in a race to break out from being a forever-niche energy source.
How Far Can It Go?
The geothermal technology flywheel is getting close to being self-sustaining. Commercial closed-loop projects should be operational within the next 2-3 years. They will spark copycats if they are successful. Companies pursuing other technologies can switch immediately by changing vendors and their well design, similar to how many solar thermal projects converted to photovoltaics.
Talent isn't an issue in North America or Europe because the oil industry constantly trains and sheds workers due to the market cycle. Geothermal companies can hoover up the laid-off and disillusioned. It could be an issue in the areas of the world with thin oilfield service markets.
Process heat should be more profitable than electricity, but companies love touting electricity because of the large addressable market. Electricity is trendy! There should be demand for repeatable, low-risk architectures in either application, but electricity will require subsidies or favorable market rules in most locations for now. I'd recommend caution to any lobbyists trying to alter electricity markets to favor zero carbon, dispatchable power plants with >90% percent capacity factors and onsite fuel. Other technologies might find geothermal power plants producing $50/MWh electricity with <2-year construction times hard to beat!
Having the chance to get in the game and hone your craft is all you can ask for. Geothermal looks like it will get that chance. That could drop the cost of delivering process heat precipitously while the power plant portion of capital expenditure would quickly dominate electricity production cost. Developers and service companies will incrementally improve depth and temperature to increase the addressable market. Competition looms from other carbon-free process heat technologies and renewables firming techniques. Only time will tell if geothermal can gain a foothold in providing heat or earn a double-digit share of the electricity market.