Balancing the Grid at Millisecond Time Scales
Power grids must maintain frequency at all times.
Frequency Control
Electric grids generally use alternating current (AC). A grid's frequency is a measure of how fast voltage is alternating between positive and negative. A steady frequency is critical to prevent damage to electrical equipment and maintain grid stability.
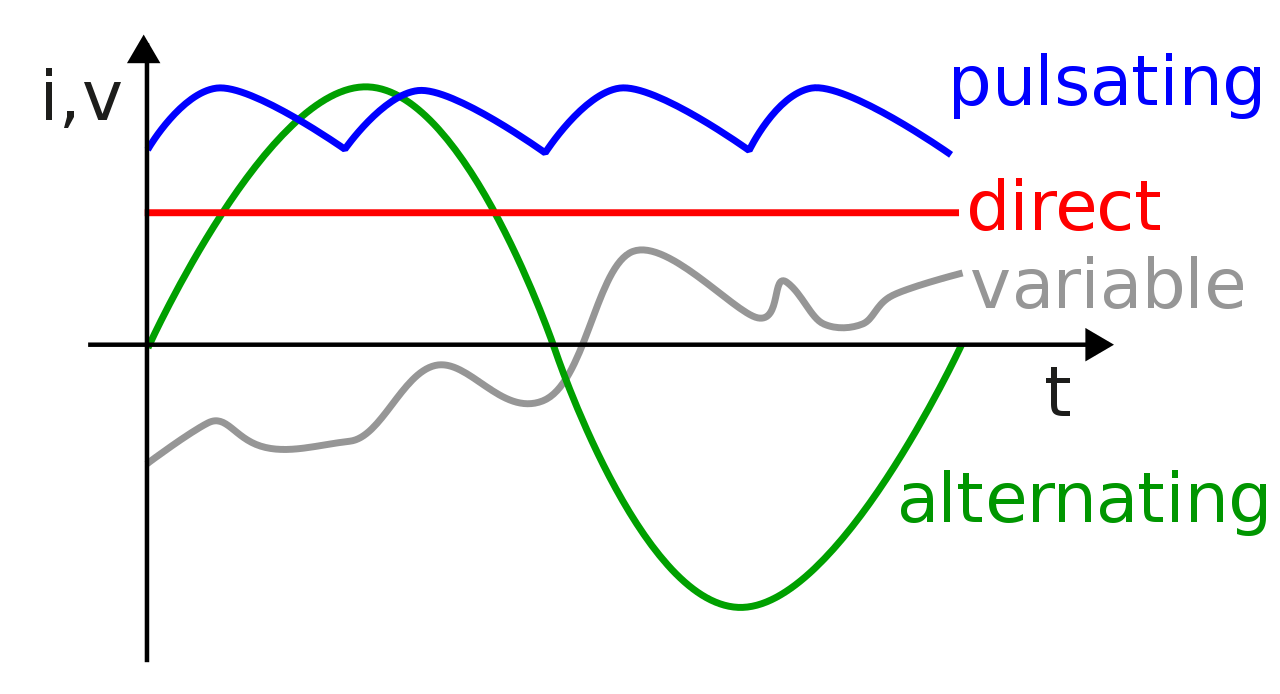
V is for voltage, i is for current. Source: Wikipedia
AC electricity frequency is set by how fast generators spin. Hydro, geothermal, nuclear, gas, oil, and coal power plants create work to spin a generator to produce electricity. Power plant generators turn at the same speed to remain in sync.
At every moment, power plants must produce the exact amount of electricity consumers use. The load on other plants increases if a power plant trips offline. Generators slow down under increased load. The spinning mass in the machinery slows, releasing energy and providing a rapid response to balance generation and load. The slower speed lowers frequency, and within power plants, more power is sent to turbines to return to normal conditions. The same is true in reverse, with rotating machinery absorbing energy as RPMs increase. Using inertia to provide fine-tuning is expensive because each generator has limited energy stored via spinning mass. More plants have to run at lower outputs to maintain enough safety margin. Increasing production from running plants or starting plants from cold conditions is too slow. The smaller the grid and the more concentrated power plants are, the more expensive frequency regulation is.
Adding Variable Renewables
Conservative grid operators initially thought the maximum penetration for solar and wind would be less than 5% at any time because of frequency control concerns. Solar and wind are asynchronous, and grid operators could not predict future output from weather forecasts. Adding more asynchronous solar and wind generation would force existing power plants to operate at lower capacity to maintain frequency control.
Today, solar and wind regularly hit over 50% penetration on modern grids. Grid operators are better at predicting renewables, and some solar and wind production can be held in reserve to make up for disruptions. But the real advance in frequency control is lithium-ion batteries.
The Cavalry Arrives
Batteries are more efficient at frequency regulation than spinning generators. While inertia response dissipates in a blink, batteries can run at max output for as long as they have a charge. A study in Ireland found that 1 MW of batteries provided the same amount of frequency regulation as 10 MW of traditional generators.
Where grid operators create frequency regulation markets, batteries quickly dominate. Many of the earliest US utility-scale battery deployments came in PJM's frequency market, where they were competitive even at 5x today's battery costs. In South Australia, frequency regulation was a sizable portion of customer electricity bills until a large battery installation crashed the price of frequency regulation by 90%.
The original "killer app" for batteries is frequency regulation, and its success lowers electricity costs by allowing traditional generators to run under more efficient operating conditions.
Consistently Meeting Average Power Demand
Electricity demand has a daily and seasonal rhythm that grids must meet.
Variability vs. Unpredictability
Grid planners can deal with variability as long as it is predictable. Unpredictability, from wind speed or unscheduled maintenance at a thermal power plant, causes headaches.
Wind Power
Wind power provides ultra-cheap electricity, but it is harder to predict the future output.
Power Laws Drive Unpredictability
The output of a traditional wind turbine increases 8x when the wind speed doubles. The energy contained in a moving air mass increases at the square of velocity. Doubling wind speed gives a 4x increase. The faster airspeed means twice the mass of air passes the turbine, doubling the energy again (4 * 2 = 8). An 11 mph wind produces 30% more output than a 10 mph wind. Predicting future wind speed at such granularity is challenging.
Geography Helps More Than Storage
Batteries usually have 1-10 hours of storage and do not help if calm weather lowers wind electricity output for days or weeks. Adding more turbines in one location is not helpful, as the speed effect on production is too steep.
Spreading wind farms across regions helps reduce the probability of extended windless periods the most. There is an adage that the wind is blowing somewhere. Increasing wind market share requires an extensive buildout of electricity transmission infrastructure. Transmission projects on land see heavy local opposition. Undersea projects down the US Atlantic seaboard or across the Baltic Sea face less opposition but are more expensive.
Without adequate transmission infrastructure, the market self-regulates wind penetration. Wind farms produce together, crashing local wholesale prices, and can't take advantage of higher prices during lower wind periods. It isn't uncommon to see typical electricity prices in Oklahoma City but zero or negative prices 100 miles away in Western Oklahoma.
When the Wind Doesn't Blow
Resources pairing with wind need cheap, high volume storage and inexpensive, fast ramping power output. Two energy sources meet those conditions - natural gas and hydropower.
Hydropower plants can quickly ramp their output up and down at a low cost. Because reservoir levels often limit yearly production, wind power can displace hydro when the wind blows and extend the hydro resource. Hydropower is limited by geography, providing around 10% of US electricity. In Europe, Germany and Norway recently finished an undersea cable project named NordLink that can move 1.4 GW of electricity bi-directionally. German wind will conserve Norway's hydro reservoirs while Norway provides hydroelectricity on calm days.
Natural gas-fired electricity is the primary backup in wind-heavy regions like the US great plains and other parts of Europe. States like Oklahoma and Kansas get 30%+ of their electricity from wind, balanced by natural gas turbines. Wind electricity has a similar share in Germany. In the United States, with inexpensive shale gas, this isn't a concern. It is not as convenient in Europe, where half its gas comes from Russia.
Coal-fired power plants do not cycle as well as natural gas power plants, raising costs. Coal also emits significantly more air pollution, making it undesirable to use in general.
It makes sense to run nuclear plants flat out all the time. There is little reason to pair nuclear with wind electricity.
Solar PV
Unlike wind, solar is predictable. The full-year output can be estimated within a half percent, making it one of the most predictable electricity generation technologies.
Solar panel electricity production is proportional to solar intensity, meaning there are no wild swings based on small changes in sunlight. Overcast days can be predicted in advance, allowing grid operators to schedule other resources.
Even on cloudy days, solar panels produce a significant amount of electricity. Solar panels have gotten so cheap that a facility owner can add extra panels to produce the desired output. On sunny days, excess electricity can charge batteries. The combination of adding panels and batteries makes the solar output "near firm," like a thermal power plant. As the cost of panels and batteries decreases, farm owners can add more to make solar electricity more reliable and increase market share. I covered the details of this process in a previous blog post.
The Achilles heels of solar availability are powering electric space heating and high latitudes. Shorter winter days and overnight lows require excessive amounts of panels and batteries to maintain reliability. I covered alternative options in a post on heating. The latitude problem is an issue for Europe. Maine is almost as sunny as Spain. Places like Germany and Scotland are downright gloomy in comparison.
Given the near-term cost trajectories, reaching 40%-50% market share in the US might be the market equilibrium. The long-term market share could rise even higher.
Short-Term Storage
Daily Cycling
Daily cycling is an emerging sweet spot for modern batteries. Batteries charge at times with low power prices and sell into the grid when demand and prices are high. Depending on the grid and season, batteries can get 1-2 cycles per day.
Average Daily PJM Load Curves by Season Source: PJM Learning Center
Batteries help solar and wind farms in two primary ways. First, they shift electricity to higher earning hours. Second, batteries smooth electricity output through changes in wind speed or sunlight. They also allow higher utilization of grid connections.
Batteries make baseload plants more efficient as well. Instead of needing the capacity to meet peak daily demand, the system only needs analog plants to meet average daily demand.
Steady is better.
Materials Availability and Future Costs
The cost of these batteries is plummeting. Material constraints are not an issue because storage batteries do not require top-tier energy density.
A few gigawatt-hours of Tesla Megapacks cost around $300/kWh as of late 2021. The battery cells probably cost less than $100/kWh. There are still high costs for packaging, cooling, inverters, transportation, and installation. Plus, cells are in short supply due to automotive demand leading to opportunity costs. The cost per cycle is ~$100/MWh, making storage competitive for frequency control and daily cycling in high-cost markets like Hawaii and California. The cycle cost is how much it costs to charge and discharge the battery each time, not accounting for purchasing electricity. [1]
Maturing storage products will drop the cost significantly. First, storage products have been using automotive battery cells. Most manufacturers plan to switch from lithium, nickel, and cobalt cells to lithium iron phosphate (LFP) cells. Not only are LFP cells cheaper, but they need less cooling and safety equipment because they are inherently safer. The battery capacity degrades much slower, providing more cycles. Iron and phosphate are cheap and available compared to cobalt and nickel. Lithium is not rare, but demand is growing so fast mining may take a few years to catch up. The learning curve for batteries is around a 20% cost reduction for every doubling of cumulative capacity. We could easily see five doublings in the next ten years, reducing costs by over 70%. Half of the five doublings volume is already in the California grid operator's interconnection queue. Cycle costs would fall to $30/MWh, making batteries competitive for daily cycling in almost every market, driving further adoption.
In the long term, even cheaper chemistries may emerge. CATL, the largest battery cell maker, recently announced the introduction of a sodium-ion battery. Its raw materials are sodium, iron, carbon, nitrogen, and silicon. So mostly dirt and air. Long-term costs could fall to $40/kWh. Cycle costs could be as low as $15/MWh with daily cycling. 24/7 solar + storage would be cheaper than running existing analog power plants in meeting average daily demand.
Batteries will be ubiquitous.
Multi-Day Battery Storage
Widely deployed lithium-ion batteries typically have between 1 hour and 10 hours of charge. Entrepreneurs are working to create batteries with 10-100 hours of storage to handle multi-day events.
It isn't clear that there is even a market for batteries with these attributes. The poster child of this category, Form Energy, has an aspirational goal of a 100-hour iron-air battery that costs $20/kWh. A 100 kWh, 1 kW battery would cost $2000/kW. Tripling the number of solar panels on a utility-scale farm can maintain power output on cloudy days at $1200-$1500/kW. That price is likely to fall over time. The batteries could alternatively compete against gas turbines during peak events like heat waves. A simple cycle turbine costs $700/kW, and its fuel costs are minimal when rarely running.
In response, Form Energy has developed complicated modeling software to showcase its value. It shows the UK will have multi-day events where transmission line congestion forces curtailment of wind-generated electricity. Form's batteries store the otherwise wasted power and sell it when transmission capacity is available. If the battery constantly charges and discharges over a 30-year life, the average undiscounted cycle cost would be under $30/MWh.
Longer-duration batteries might have a place in countries with ultra-low interest rates, high wind penetration, and poor solar resources.
Handling Peak Demand
The "Peaker" Problem
Grid operators size capacity for the absolute worst-case moment because the grid must balance at all times. Some power plants might run one hour per year. The cost of operating the last megawatts is astronomical. To try and reduce these costs, most rarely run power plants are simple cycle gas turbines that cost $700/kW instead of the $2500-$8000/kW that non-gas baseload power plants cost. Fuel cost is inconsequential compared to the capital cost in this application.
The "Smart Grid"
Smart meters, smart thermostats, smart dishwashers, smart water heaters, and more exist that can adjust energy usage based on electricity prices or pre-programmed behavior. Because the cost of the marginal kWh at peak demand is so high, the business case for shaving demand should be strong. The impact has been disappointing.
Oklahoma Gas + Electric has one of the most ambitious smart-grid programs. During the summer, customers can sign up for Smart Hours. They get a free programmable thermostat and half-price electricity during off-peak hours, around $0.05/kWh. On hot days, their electricity price during the 2 PM-7 PM period can increase up to $0.47/kWh. The thermostat pre-cools the house in the morning then turns the AC off until 7 PM, unless customers override it. Judging by social media chatter, it is usually a sweat fest to save a couple of hundred bucks per season. In a pilot study, customers often gave up before 7 PM limiting the peak savings. OGE found the full rollout reduces peak demand by 72 MW. OGE's generation capacity is over 6000 MW. The original goal was to eliminate the construction of 300 MW of new peaker plants.
While peakers may be inefficient, electricity bills are a small part of most customers' spending, and their preference is to pay higher rates for always available electricity. The long-term approach to the smart grid should focus on new sources of demand like electric cars and should incentivize customers to reduce usage during critical events that threaten grid stability. They might take a $100 payment to change the thermostat for two days once every few years, but they won't sweat it out 30 evenings a year to save the same.
Long-Term Storage
Wacky ideas for long-term energy storage abound. Let's see why adoption is low.
The Pretenders
While the ideas are sound, high costs and limited deployment opportunities doom these concepts.
Low Cost, but Niche
Pumped storage needs special geographical features. Thermal storage probably needs to share infrastructure to be competitive.
The Contenders
Hydrogen
Hydrogen is the hype man of long-term storage. It pains me to put it in the contender category.
Hydrogen fails to gain adoption in applications because it has horrible volumetric density and damages steel. Industry uses hydrogen in many processes, but chemical plants consume hydrogen without storing or transporting it.
If electrolyzer costs drop rapidly, which they could, hydrogen storage might work where geological storage is feasible. A storage site would produce hydrogen from cheap electricity, store it in a salt cavern or reservoir, then burn it in a turbine or fuel cell to produce electricity.
Hydrogen systems have the capital cost of the electrolyzer, the storage volume, the fuel cell/turbine, and all the associated compressors, safety interlocks, and piping. Round trip efficiency is less than 50%. Transportation offsite in large quantities requires new, expensive pipelines because of the stress cracking problem.
Drop-in Fuels
Catalysts for making fuels from CO2, water, and electricity have rapidly improved over the last few years. These new processes can produce methane (natural gas), ethylene, ethanol, gasoline, diesel, heating fuel, and jet fuel.
Efficiency is lower, but the technology can dramatically reduce capital costs. There is no need for electrolyzers, and the existing hydrocarbon infrastructure is already available without modification. Costs will fall further as catalysts improve and solar + wind electricity prices fall. Hydrogen storage will benefit from falling electricity and electrolyzer costs, but building or converting storage and generation facilities will remain expensive.
Bottom Line
Long-term storage has a challenging business case. Solar + wind + batteries will eat more and more market share. Tweener storage products like 100-hour batteries could clean up even more.
Existing natural gas or hydropower plants will remain extremely cost-competitive in balancing the grid because they already exist. Falling synthetic hydrocarbon prices and more efficient power markets that utilize real-time price signals could kill whatever business case competing longer-term storage concepts have.
Utilizing Variable Sources
There are emerging sources of demand that will be more responsive to real-time electricity pricing.
Transportation
Consumers may not respond to price signals by adjusting the thermostat, but they might change their car charging behavior. Electrification of transport will dramatically increase electricity demand. The increased demand will encourage investment in new solar and wind power plants. The average electric car sold has over 200 miles of range. Most people drive less than 30 miles on a median day. Customers can delay charging for days with no loss in utility. Flexible transportation demand improves the business case for oversizing solar facilities and allows increased wind penetration.
So far, vehicle-to-grid is not a thing, but flexible charging provides huge benefits regardless.
Regulators and utilities have to put in the effort to create these markets. Transportation offers the best source of flexible demand that can lower electricity costs and improve grid reliability.
New Industrial Users
The fertilizer, refining, and steel industries can use falling hydrogen costs to replace natural gas-derived hydrogen and coal in their processes. Hydrogen storage is still a tricky proposition, so if nuclear thermal hydrogen becomes available at a price anywhere near renewables-powered low-temperature electrolysis, it will dominate industrial applications. Industrial hydrogen may or may not need the grid as a result.
Synthetic hydrocarbons will find uses in more applications than electricity storage. Heating fuel and fuel for ships and planes are potential sources of demand. Flexible calcium carbonate production (for concrete) is another possible customer. Based on how utilities currently charge industrial users for electricity, these new users will not be on the grid.
Typically, industrial users pay "demand charges" based on their peak demand in the billing period. Demand charges pay for the space reserved on an analog power plant and transmission network. Then they are charged rates for each kWh based on the average marginal cost of producing electricity from all power plants, including expensive gas and coal plants.
The business case for synthetic hydrocarbons and other lower utilization facilities relies on using only cheap, variable renewable energy when it is available. Demand charges and average rates kill the business case. The alternative is private solar and wind grids with minimal batteries where electricity might cost under $20/MWh.
Having these facilities integrated into the grid could offer an electricity generation buffer. Grid operators can't even designate a place to site a facility without an expensive, years-long process. Utilities will have to develop new rate structures, and grid operators will have to overhaul their interconnection processes to earn this source of grid flexibility,
Our Likely Future
We can draw several conclusions from this analysis.
-
Grid frequency control is a solved problem because batteries are a leap in technology
-
Meeting average power demand in high renewable penetration grids is easy except where solar resources are weak combined with high wind penetration. Sorry, Northwest Europe. Solar might be cheap enough to win before they can finish a traditional nuclear power plant, though.
-
Peak events are still best met by existing natural gas or hydropower plants, but new sources of interruptible demand can ease the burden. Synthetic fuel or direct air capture can solve emissions, especially as power plant utilization falls and makes the natural gas price less consequential.
In the US, my best guess for the medium term market share on the grid:
- Solar: 40%-50%
- Wind: 20%
- Natural Gas: 10%-20%
- Nuclear + Hydro: 20%
As demand from transportation and other sources rises, the solar and wind share will continue to increase because current nuclear and hydro technology takes decades to build and is horrifically expensive. Gas is the wild card in that capacity exists for it to have a higher share. Low wholesale prices will likely push it out of baseload to higher-priced hours. Coal is going to zero for economic reasons alone. Solar will eat more gas, nuclear, and hydro market share unless other competitors see tremendous improvement.
New industrial users will rarely connect to the grid, implying substantial volumes of non-grid solar and wind. Grid electricity sales and solar + wind share will rise further if utility rate structures change.
The more rigid the institutions around our electricity system remain, the higher the price customers will pay and the lower the reliability they will receive.
[1] Cycle Cost = ((CAPEX / Lifetime Cycles) + OPEX) * Discounting Factor
The Three Horseman of Grid Stability
2021 November 5 Twitter Substack See all postsGrid operators must balance the grid at the millisecond level, the daily level, and during peak events.
Balancing the Grid at Millisecond Time Scales
Power grids must maintain frequency at all times.
Frequency Control
Electric grids generally use alternating current (AC). A grid's frequency is a measure of how fast voltage is alternating between positive and negative. A steady frequency is critical to prevent damage to electrical equipment and maintain grid stability.
V is for voltage, i is for current. Source: Wikipedia
AC electricity frequency is set by how fast generators spin. Hydro, geothermal, nuclear, gas, oil, and coal power plants create work to spin a generator to produce electricity. Power plant generators turn at the same speed to remain in sync.
At every moment, power plants must produce the exact amount of electricity consumers use. The load on other plants increases if a power plant trips offline. Generators slow down under increased load. The spinning mass in the machinery slows, releasing energy and providing a rapid response to balance generation and load. The slower speed lowers frequency, and within power plants, more power is sent to turbines to return to normal conditions. The same is true in reverse, with rotating machinery absorbing energy as RPMs increase. Using inertia to provide fine-tuning is expensive because each generator has limited energy stored via spinning mass. More plants have to run at lower outputs to maintain enough safety margin. Increasing production from running plants or starting plants from cold conditions is too slow. The smaller the grid and the more concentrated power plants are, the more expensive frequency regulation is.
Adding Variable Renewables
Conservative grid operators initially thought the maximum penetration for solar and wind would be less than 5% at any time because of frequency control concerns. Solar and wind are asynchronous, and grid operators could not predict future output from weather forecasts. Adding more asynchronous solar and wind generation would force existing power plants to operate at lower capacity to maintain frequency control.
Today, solar and wind regularly hit over 50% penetration on modern grids. Grid operators are better at predicting renewables, and some solar and wind production can be held in reserve to make up for disruptions. But the real advance in frequency control is lithium-ion batteries.
The Cavalry Arrives
Batteries are more efficient at frequency regulation than spinning generators. While inertia response dissipates in a blink, batteries can run at max output for as long as they have a charge. A study in Ireland found that 1 MW of batteries provided the same amount of frequency regulation as 10 MW of traditional generators.
Where grid operators create frequency regulation markets, batteries quickly dominate. Many of the earliest US utility-scale battery deployments came in PJM's frequency market, where they were competitive even at 5x today's battery costs. In South Australia, frequency regulation was a sizable portion of customer electricity bills until a large battery installation crashed the price of frequency regulation by 90%.
The original "killer app" for batteries is frequency regulation, and its success lowers electricity costs by allowing traditional generators to run under more efficient operating conditions.
Consistently Meeting Average Power Demand
Electricity demand has a daily and seasonal rhythm that grids must meet.
Variability vs. Unpredictability
Grid planners can deal with variability as long as it is predictable. Unpredictability, from wind speed or unscheduled maintenance at a thermal power plant, causes headaches.
Wind Power
Wind power provides ultra-cheap electricity, but it is harder to predict the future output.
Power Laws Drive Unpredictability
The output of a traditional wind turbine increases 8x when the wind speed doubles. The energy contained in a moving air mass increases at the square of velocity. Doubling wind speed gives a 4x increase. The faster airspeed means twice the mass of air passes the turbine, doubling the energy again (4 * 2 = 8). An 11 mph wind produces 30% more output than a 10 mph wind. Predicting future wind speed at such granularity is challenging.
Geography Helps More Than Storage
Batteries usually have 1-10 hours of storage and do not help if calm weather lowers wind electricity output for days or weeks. Adding more turbines in one location is not helpful, as the speed effect on production is too steep.
Spreading wind farms across regions helps reduce the probability of extended windless periods the most. There is an adage that the wind is blowing somewhere. Increasing wind market share requires an extensive buildout of electricity transmission infrastructure. Transmission projects on land see heavy local opposition. Undersea projects down the US Atlantic seaboard or across the Baltic Sea face less opposition but are more expensive.
Without adequate transmission infrastructure, the market self-regulates wind penetration. Wind farms produce together, crashing local wholesale prices, and can't take advantage of higher prices during lower wind periods. It isn't uncommon to see typical electricity prices in Oklahoma City but zero or negative prices 100 miles away in Western Oklahoma.
When the Wind Doesn't Blow
Resources pairing with wind need cheap, high volume storage and inexpensive, fast ramping power output. Two energy sources meet those conditions - natural gas and hydropower.
Hydropower plants can quickly ramp their output up and down at a low cost. Because reservoir levels often limit yearly production, wind power can displace hydro when the wind blows and extend the hydro resource. Hydropower is limited by geography, providing around 10% of US electricity. In Europe, Germany and Norway recently finished an undersea cable project named NordLink that can move 1.4 GW of electricity bi-directionally. German wind will conserve Norway's hydro reservoirs while Norway provides hydroelectricity on calm days.
Natural gas-fired electricity is the primary backup in wind-heavy regions like the US great plains and other parts of Europe. States like Oklahoma and Kansas get 30%+ of their electricity from wind, balanced by natural gas turbines. Wind electricity has a similar share in Germany. In the United States, with inexpensive shale gas, this isn't a concern. It is not as convenient in Europe, where half its gas comes from Russia.
Coal-fired power plants do not cycle as well as natural gas power plants, raising costs. Coal also emits significantly more air pollution, making it undesirable to use in general.
It makes sense to run nuclear plants flat out all the time. There is little reason to pair nuclear with wind electricity.
Solar PV
Unlike wind, solar is predictable. The full-year output can be estimated within a half percent, making it one of the most predictable electricity generation technologies.
Solar panel electricity production is proportional to solar intensity, meaning there are no wild swings based on small changes in sunlight. Overcast days can be predicted in advance, allowing grid operators to schedule other resources.
Even on cloudy days, solar panels produce a significant amount of electricity. Solar panels have gotten so cheap that a facility owner can add extra panels to produce the desired output. On sunny days, excess electricity can charge batteries. The combination of adding panels and batteries makes the solar output "near firm," like a thermal power plant. As the cost of panels and batteries decreases, farm owners can add more to make solar electricity more reliable and increase market share. I covered the details of this process in a previous blog post.
The Achilles heels of solar availability are powering electric space heating and high latitudes. Shorter winter days and overnight lows require excessive amounts of panels and batteries to maintain reliability. I covered alternative options in a post on heating. The latitude problem is an issue for Europe. Maine is almost as sunny as Spain. Places like Germany and Scotland are downright gloomy in comparison.
Given the near-term cost trajectories, reaching 40%-50% market share in the US might be the market equilibrium. The long-term market share could rise even higher.
Short-Term Storage
Daily Cycling
Daily cycling is an emerging sweet spot for modern batteries. Batteries charge at times with low power prices and sell into the grid when demand and prices are high. Depending on the grid and season, batteries can get 1-2 cycles per day.
Average Daily PJM Load Curves by Season Source: PJM Learning Center
Batteries help solar and wind farms in two primary ways. First, they shift electricity to higher earning hours. Second, batteries smooth electricity output through changes in wind speed or sunlight. They also allow higher utilization of grid connections.
Batteries make baseload plants more efficient as well. Instead of needing the capacity to meet peak daily demand, the system only needs analog plants to meet average daily demand.
Steady is better.
Materials Availability and Future Costs
The cost of these batteries is plummeting. Material constraints are not an issue because storage batteries do not require top-tier energy density.
A few gigawatt-hours of Tesla Megapacks cost around $300/kWh as of late 2021. The battery cells probably cost less than $100/kWh. There are still high costs for packaging, cooling, inverters, transportation, and installation. Plus, cells are in short supply due to automotive demand leading to opportunity costs. The cost per cycle is ~$100/MWh, making storage competitive for frequency control and daily cycling in high-cost markets like Hawaii and California. The cycle cost is how much it costs to charge and discharge the battery each time, not accounting for purchasing electricity. [1]
Maturing storage products will drop the cost significantly. First, storage products have been using automotive battery cells. Most manufacturers plan to switch from lithium, nickel, and cobalt cells to lithium iron phosphate (LFP) cells. Not only are LFP cells cheaper, but they need less cooling and safety equipment because they are inherently safer. The battery capacity degrades much slower, providing more cycles. Iron and phosphate are cheap and available compared to cobalt and nickel. Lithium is not rare, but demand is growing so fast mining may take a few years to catch up. The learning curve for batteries is around a 20% cost reduction for every doubling of cumulative capacity. We could easily see five doublings in the next ten years, reducing costs by over 70%. Half of the five doublings volume is already in the California grid operator's interconnection queue. Cycle costs would fall to $30/MWh, making batteries competitive for daily cycling in almost every market, driving further adoption.
In the long term, even cheaper chemistries may emerge. CATL, the largest battery cell maker, recently announced the introduction of a sodium-ion battery. Its raw materials are sodium, iron, carbon, nitrogen, and silicon. So mostly dirt and air. Long-term costs could fall to $40/kWh. Cycle costs could be as low as $15/MWh with daily cycling. 24/7 solar + storage would be cheaper than running existing analog power plants in meeting average daily demand.
Batteries will be ubiquitous.
Multi-Day Battery Storage
Widely deployed lithium-ion batteries typically have between 1 hour and 10 hours of charge. Entrepreneurs are working to create batteries with 10-100 hours of storage to handle multi-day events.
It isn't clear that there is even a market for batteries with these attributes. The poster child of this category, Form Energy, has an aspirational goal of a 100-hour iron-air battery that costs $20/kWh. A 100 kWh, 1 kW battery would cost $2000/kW. Tripling the number of solar panels on a utility-scale farm can maintain power output on cloudy days at $1200-$1500/kW. That price is likely to fall over time. The batteries could alternatively compete against gas turbines during peak events like heat waves. A simple cycle turbine costs $700/kW, and its fuel costs are minimal when rarely running.
In response, Form Energy has developed complicated modeling software to showcase its value. It shows the UK will have multi-day events where transmission line congestion forces curtailment of wind-generated electricity. Form's batteries store the otherwise wasted power and sell it when transmission capacity is available. If the battery constantly charges and discharges over a 30-year life, the average undiscounted cycle cost would be under $30/MWh.
Longer-duration batteries might have a place in countries with ultra-low interest rates, high wind penetration, and poor solar resources.
Handling Peak Demand
The "Peaker" Problem
Grid operators size capacity for the absolute worst-case moment because the grid must balance at all times. Some power plants might run one hour per year. The cost of operating the last megawatts is astronomical. To try and reduce these costs, most rarely run power plants are simple cycle gas turbines that cost $700/kW instead of the $2500-$8000/kW that non-gas baseload power plants cost. Fuel cost is inconsequential compared to the capital cost in this application.
The "Smart Grid"
Smart meters, smart thermostats, smart dishwashers, smart water heaters, and more exist that can adjust energy usage based on electricity prices or pre-programmed behavior. Because the cost of the marginal kWh at peak demand is so high, the business case for shaving demand should be strong. The impact has been disappointing.
Oklahoma Gas + Electric has one of the most ambitious smart-grid programs. During the summer, customers can sign up for Smart Hours. They get a free programmable thermostat and half-price electricity during off-peak hours, around $0.05/kWh. On hot days, their electricity price during the 2 PM-7 PM period can increase up to $0.47/kWh. The thermostat pre-cools the house in the morning then turns the AC off until 7 PM, unless customers override it. Judging by social media chatter, it is usually a sweat fest to save a couple of hundred bucks per season. In a pilot study, customers often gave up before 7 PM limiting the peak savings. OGE found the full rollout reduces peak demand by 72 MW. OGE's generation capacity is over 6000 MW. The original goal was to eliminate the construction of 300 MW of new peaker plants.
While peakers may be inefficient, electricity bills are a small part of most customers' spending, and their preference is to pay higher rates for always available electricity. The long-term approach to the smart grid should focus on new sources of demand like electric cars and should incentivize customers to reduce usage during critical events that threaten grid stability. They might take a $100 payment to change the thermostat for two days once every few years, but they won't sweat it out 30 evenings a year to save the same.
Long-Term Storage
Wacky ideas for long-term energy storage abound. Let's see why adoption is low.
The Pretenders
Compressed Air
Locomotives driving rocks up hills
Cranes building or deconstruction block towers
While the ideas are sound, high costs and limited deployment opportunities doom these concepts.
Low Cost, but Niche
Pumped Water Storage
A hydro reservoir where you pump water back up into the lake when electricity is cheap.
Thermal Storage
The idea is to heat rocks or melt salt then recover the heat with a turbine.
Pumped storage needs special geographical features. Thermal storage probably needs to share infrastructure to be competitive.
The Contenders
Hydrogen
Hydrogen is the hype man of long-term storage. It pains me to put it in the contender category.
Hydrogen fails to gain adoption in applications because it has horrible volumetric density and damages steel. Industry uses hydrogen in many processes, but chemical plants consume hydrogen without storing or transporting it.
If electrolyzer costs drop rapidly, which they could, hydrogen storage might work where geological storage is feasible. A storage site would produce hydrogen from cheap electricity, store it in a salt cavern or reservoir, then burn it in a turbine or fuel cell to produce electricity.
Hydrogen systems have the capital cost of the electrolyzer, the storage volume, the fuel cell/turbine, and all the associated compressors, safety interlocks, and piping. Round trip efficiency is less than 50%. Transportation offsite in large quantities requires new, expensive pipelines because of the stress cracking problem.
Drop-in Fuels
Catalysts for making fuels from CO2, water, and electricity have rapidly improved over the last few years. These new processes can produce methane (natural gas), ethylene, ethanol, gasoline, diesel, heating fuel, and jet fuel.
Efficiency is lower, but the technology can dramatically reduce capital costs. There is no need for electrolyzers, and the existing hydrocarbon infrastructure is already available without modification. Costs will fall further as catalysts improve and solar + wind electricity prices fall. Hydrogen storage will benefit from falling electricity and electrolyzer costs, but building or converting storage and generation facilities will remain expensive.
Bottom Line
Long-term storage has a challenging business case. Solar + wind + batteries will eat more and more market share. Tweener storage products like 100-hour batteries could clean up even more.
Existing natural gas or hydropower plants will remain extremely cost-competitive in balancing the grid because they already exist. Falling synthetic hydrocarbon prices and more efficient power markets that utilize real-time price signals could kill whatever business case competing longer-term storage concepts have.
Utilizing Variable Sources
There are emerging sources of demand that will be more responsive to real-time electricity pricing.
Transportation
Consumers may not respond to price signals by adjusting the thermostat, but they might change their car charging behavior. Electrification of transport will dramatically increase electricity demand. The increased demand will encourage investment in new solar and wind power plants. The average electric car sold has over 200 miles of range. Most people drive less than 30 miles on a median day. Customers can delay charging for days with no loss in utility. Flexible transportation demand improves the business case for oversizing solar facilities and allows increased wind penetration.
So far, vehicle-to-grid is not a thing, but flexible charging provides huge benefits regardless.
Regulators and utilities have to put in the effort to create these markets. Transportation offers the best source of flexible demand that can lower electricity costs and improve grid reliability.
New Industrial Users
The fertilizer, refining, and steel industries can use falling hydrogen costs to replace natural gas-derived hydrogen and coal in their processes. Hydrogen storage is still a tricky proposition, so if nuclear thermal hydrogen becomes available at a price anywhere near renewables-powered low-temperature electrolysis, it will dominate industrial applications. Industrial hydrogen may or may not need the grid as a result.
Synthetic hydrocarbons will find uses in more applications than electricity storage. Heating fuel and fuel for ships and planes are potential sources of demand. Flexible calcium carbonate production (for concrete) is another possible customer. Based on how utilities currently charge industrial users for electricity, these new users will not be on the grid.
Typically, industrial users pay "demand charges" based on their peak demand in the billing period. Demand charges pay for the space reserved on an analog power plant and transmission network. Then they are charged rates for each kWh based on the average marginal cost of producing electricity from all power plants, including expensive gas and coal plants.
The business case for synthetic hydrocarbons and other lower utilization facilities relies on using only cheap, variable renewable energy when it is available. Demand charges and average rates kill the business case. The alternative is private solar and wind grids with minimal batteries where electricity might cost under $20/MWh.
Having these facilities integrated into the grid could offer an electricity generation buffer. Grid operators can't even designate a place to site a facility without an expensive, years-long process. Utilities will have to develop new rate structures, and grid operators will have to overhaul their interconnection processes to earn this source of grid flexibility,
Our Likely Future
We can draw several conclusions from this analysis.
Grid frequency control is a solved problem because batteries are a leap in technology
Meeting average power demand in high renewable penetration grids is easy except where solar resources are weak combined with high wind penetration. Sorry, Northwest Europe. Solar might be cheap enough to win before they can finish a traditional nuclear power plant, though.
Peak events are still best met by existing natural gas or hydropower plants, but new sources of interruptible demand can ease the burden. Synthetic fuel or direct air capture can solve emissions, especially as power plant utilization falls and makes the natural gas price less consequential.
In the US, my best guess for the medium term market share on the grid:
As demand from transportation and other sources rises, the solar and wind share will continue to increase because current nuclear and hydro technology takes decades to build and is horrifically expensive. Gas is the wild card in that capacity exists for it to have a higher share. Low wholesale prices will likely push it out of baseload to higher-priced hours. Coal is going to zero for economic reasons alone. Solar will eat more gas, nuclear, and hydro market share unless other competitors see tremendous improvement.
New industrial users will rarely connect to the grid, implying substantial volumes of non-grid solar and wind. Grid electricity sales and solar + wind share will rise further if utility rate structures change.
The more rigid the institutions around our electricity system remain, the higher the price customers will pay and the lower the reliability they will receive.
[1] Cycle Cost = ((CAPEX / Lifetime Cycles) + OPEX) * Discounting Factor